
With Corteva Agriscience’s unique gene-editing know-how, researchers fine-tune crops for better performance.
Gina Zastrow-Hayes speaks with the fluid precision you’d expect from someone who edits genes for a living, and with that facility, she demystifies the complex biological processes she and her team harness to improve the performance of the staple crops farmers rely on.
At the Corteva Agriscience Biotechnology Genome Center of Excellence, where she is the director, Zastrow-Hayes is leading a group of scientists and researchers who “make small modifications in very, very specific locations in the genome” of plants to accelerate the natural variations plant breeders rely on to coax beneficial traits from plants like canola, wheat, corn, and more.
She relies on CRISPR-Cas – an A-list celebrity among enzymes – for her delicate stitchwork, but “that is just one tool in the gene-editing toolbox. We need a host of enzymes to access different areas of the genome.”

Gina Zastrow-Hayes is Director at the Biotechnology Genome Center of Excellence at Corteva Agriscience.
Source: Corteva AgriscienceWith those tools, she and her colleagues introduce fine-grained changes “exactly where we want to make a modification to the genome,” she explains. They are “doing what natural variation already does to the plant” and accelerating the development of new varieties that improve yield, bolster nutrition, increase disease resistance, or solve the myriad of other problems farmers face.
Putting evolution on fast-forward
Zastrow-Hayes makes it clear that her work isn’t all that different from that which breeders have done since the
dawn of agriculture. Ten thousand years ago (give or take a millennium), native people in what is now Mexico began the arduous process of using breeding to turn teosinte, a wild grass, into maize. In the eons since, humans have continued to crossbreed corn to amplify certain traits like yield and disease resistance, and those improvements have formed the backbones of family farms and the foundations of seed companies. Pioneer® brand seed corn, for example, made its name by supplying farmers with superior hybrid seed corn created through crossbreeding.
Today, selective breeding and crossbreeding are powerful precision tools for producing better crops, and while they’re faster and more accurate than they have ever been before, gene editing gives these age-old practices a shot of pure nitro.
Isolating and propagating a desired trait through conventional means can only proceed step-by-step, rolling forward one season at a time. It goes by nature’s pace, and scientists have no assurance that they’ll be able to bring forward the new trait farmers need. Gene editing takes much of the guesswork out of the process.
From when a researcher says ‘go’ and has a target of interest to when we can deliver a plant to the greenhouse is typically about five months.”
Corteva Agriscience’s vast library of elite germplasm, their proprietary enzymatic tools, and their skill in transformation combine to make that neck-snapping speed possible.
Transcription at work
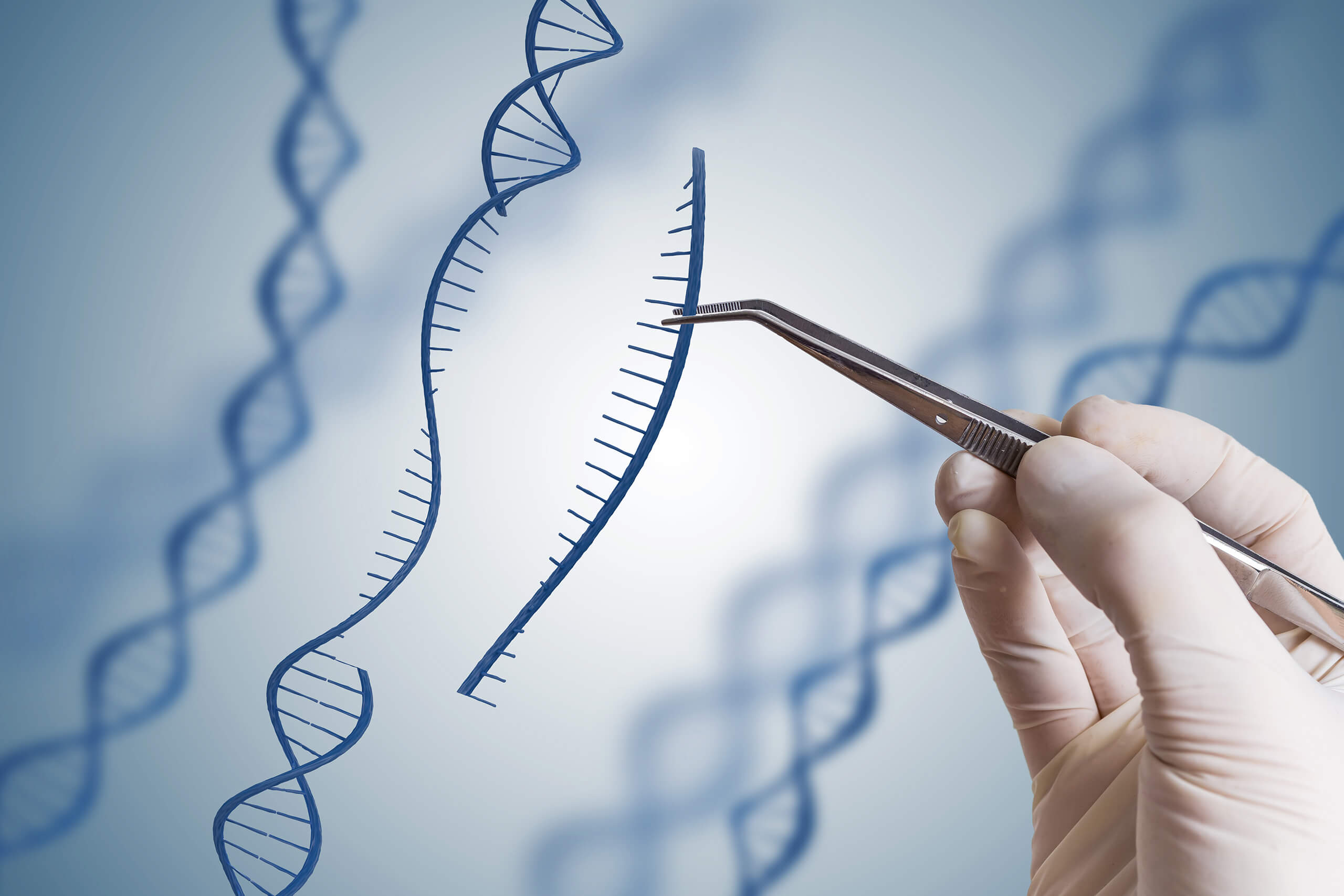
Scientists make precise edits at very specific locations in the genome.
Source: Getty ImagesThe whole gene-editing process begins with the needs of farmers. Once Corteva has identified a new attribute that would improve a crop and solve a problem for farmers, Zastrow-Hayes and her team get to work. “It’s critical that we have a deep knowledge about and understanding of the genome,” she says.
Not only do researchers have to know how to find the genes that encode the target attribute, but they also need to know which of the surrounding genes regulate the expression of that sequence. This allows them to make “intelligent decisions about where to make edits,” as Zastrow-Hayes puts it, and where to place the new snippet of DNA they’ve collected, taking care to avoid impacts to or from any surrounding regulatory elements.
Prior techniques were essentially random in where they placed target sequences. Now, though, researchers employ the CRISPR-Cas “machinery,” as Zastrow-Hayes calls it, to execute a complicated genetic dance, and this is where things get a little complicated. CRISPR-Cas consists of two parts: a strand of guide RNA that directs the machinery to the target location in the DNA and CRISPR-Cas, the enzyme that snips through both strands of a DNA pair.
When the CRISPR-Cas complex arrives at the section of DNA targeted by the researcher, it binds to the site, unwinds the DNA double helix, and pairs the guide RNA to one strand of the unwound DNA. Then the CRISPR-Cas enzyme cuts through both strands of the DNA, which the cell then repairs.
Scientists can use this mechanism to disrupt an existing gene by removing several of the nucleotides that compose it. They can also delete a gene entirely by making two such cuts, one on either side of its genetic sequence.
Farmers will benefit from a third use too: template-based editing. Researchers use CRISPR-Cas twice: first to make a double-strand break and then to insert a new segment of DNA bound to the guide RNA that came from elsewhere. For transgenic modifications, the new strand comes from a different organism, but in gene editing, such as Zastrow-Hayes is doing, the target sequence originates in a different example of the same plant, bringing the attributes of one variety together with a specific addition from another.
While still some years away from being able to improve profits for farmers, these new plants are already being tested in the field. Corteva is also expanding the application of its gene-editing tools and germplasm library through partnerships, working with organizations such as the Bill & Melinda Gates Foundation, the International Maize and Wheat Improvement Center (CIMMYT), and others to improve the resistance of maize plants to lethal necrosis in Africa, control parasitic plants in sorghum, reduce rancidity in pearl-millet flour, and enable a new soybean cropping system, improving food security and farmers’ livelihoods.
Market-led innovation
It helps to have a clear target in mind when deciding where to make changes and to which plant to make them, especially given the breadth of possibility inherent in gene editing. And for that, Corteva turns to what farmers have been requesting – things like “protecting the crop they have planted, reducing risk, helping provide stability, and improving yields.”
Canola is a perfect example. Current varieties of the plant have a preset pod-breaking point – a dehiscence zone – that can open up before harvest. That pod shatter can cause between 20% and 50% yield loss for a farmer. Using CRISPR-Cas, Zastrow-Hayes’s team found a specific genetic variation that prevents premature pod shatter. Controlling pod shatter can dramatically decrease yield loss for farmers.
The Corteva Biotechnology Genome Center of Excellence is also working on co-locating disease-resistance genes in corn to combat northern leaf blight, southern rust, gray leaf spot, and anthracnose stalk rot, all in one variety. Those four diseases slashed North American corn yields by over 318 million bushels in 2021 and hurt farmers’ bottom lines.
“The more modes of action against disease that we can deliver, the better stability our products have and the greater longevity and lower chance for resistance,” according to Zastrow-Hayes. “We wanted to deliver not only more modes of action, but also more potential coverage for multiple diseases at one time.”
While still some years away from being able to improve profits for farmers, these new plants are already being tested in the field. Corteva is also expanding the application of its gene-editing tools and germplasm library through partnerships, working with organizations such as the Bill & Melinda Gates Foundation, the International Maize and Wheat Improvement Center (CIMMYT), and others to improve the resistance of maize plants to lethal necrosis in Africa, control parasitic plants in sorghum, reduce rancidity in pearl-millet flour, and enable a new soybean cropping system, improving food security and farmers’ livelihoods.
A faster future
“These new tools have allowed us to deliver new products at a speed we’ve never been able to before,” says Zastrow-Hayes. The regulatory environment is key in moving these innovations along with alacrity; some officials have chosen not to regulate gene-edited crops as transgenics, at least in the United States.
Notwithstanding the dramatic increases in the research timeline, it still takes a few years to get a major new plant variety to market, but it’s that far-off future that Corteva thinks about today. Zastrow-Hayes believes gene editing will play a critical role in “renewable fuel and oils concepts by editing our crops to better fit those marketplaces, tailoring the composition of the seed to meet the downstream integrated market.”
And that means farmers can look forward to new revenue streams from the same acres. Bill Gates is reported to have said, “Most people overestimate what they can do in one year and underestimate what they can do in 10 years.” Regardless of who originated that aphorism, it’s one that resonates with Zastrow-Hayes as she surveys the future: “With gene editing 10 years ago, I couldn’t imagine the future we are in right now. And I could not be more excited about the future in 10 more years.”